Ngā Kawepūrongo
News
Insights, GP profiles, medical opinion and more.
-
June 2025
June 4, 2025
-
Budget 2025 misses opportunity to give primary care a leading role in addressing and improving healthcare in Aotearoa
May 25, 2025
-
Enter the 2025 Greg Judkins Poetry Competition
May 23, 2025
-
Budget 2025 opinion: We don't need to create a new health service, we need to invest in the current one
May 21, 2025
Categories
Media-Releases
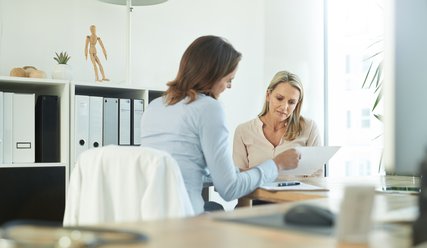
23 June 2025 | Media releases
Recognition of the GP workforce’s value to communities welcomed in funding announcement
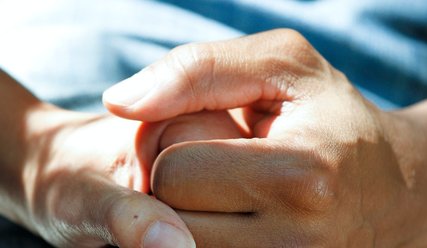
25 May 2025 | Media releases
Budget 2025 misses opportunity to give primary care a leading role in addressing and improving healthcare in Aotearoa
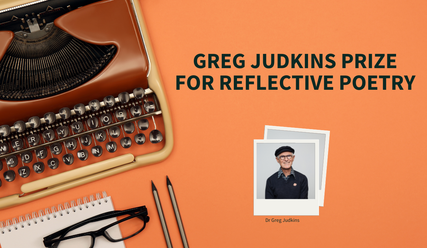
23 May 2025 | Media releases
Enter the 2025 Greg Judkins Poetry Competition
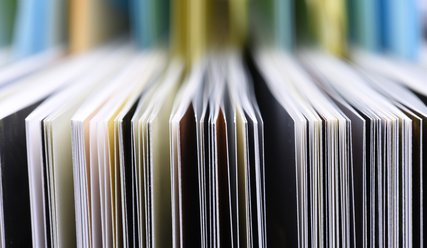
14 May 2025 | Media releases
Funding round opens for research benefitting general practice
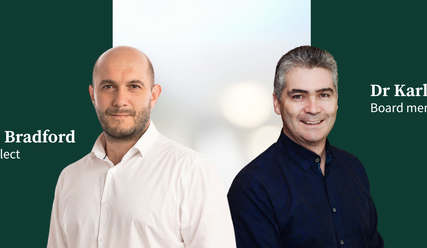
24 April 2025 | Media releases
College of GPs announces new President and Board member
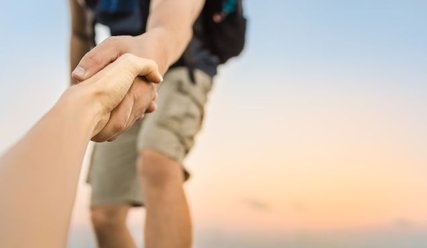
4 March 2025 | Media releases
Primary care funding a positive step in the right direction, says College of GPs
Upcoming events
See all eventsST FRANCIS RETREAT CENTER 50 HILLSBOROUGH ROAD HILLSBOROUGH AUCKLAND, 1042 NEW ZEALAND | CONTINUING MEDICAL EDUCATION
The “Billings Ovulation Method™: Natural Fertility Seminar
11 July 2025 - 12 July 2025
9:00 AM - 5:00 PM
This is a 2-day seminar where doctors will engage in a stimulating and coherent course designed to introduce the fundamentals and scientific understanding of the Billing’s Ovulation Method™.
View event4 KAHU ROAD RICCARTON CHRISTCHURCH, | CONTINUING MEDICAL EDUCATION
Mindfulness for Medical Practitioners
14 July 2025 - 08 September 2025
7:00 PM - 9:15 PM
This comprehensive 8-week Mindfulness-Based Stress Reduction (MBSR) program is specifically designed for medical practitioners.
View eventCME planning, cultural insight and self-care workshop
16 July 2025 - 16 July 2025
8:30 AM - 3:00 PM
Refresh, revitalize and get Ahead: Your ultimate CME planning and self-care workshop.
View eventMore recent articles
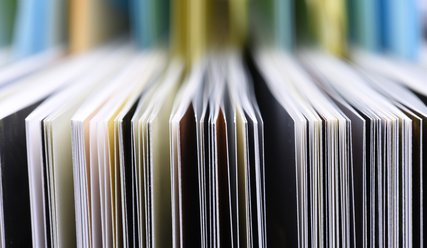
14 May 2025 | Media releases
Funding round opens for research benefitting general practice
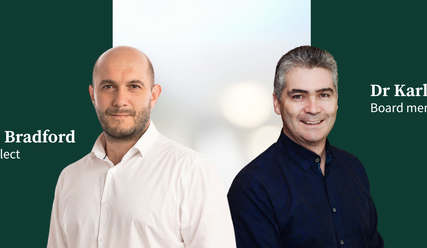
24 April 2025 | Media releases
College of GPs announces new President and Board member
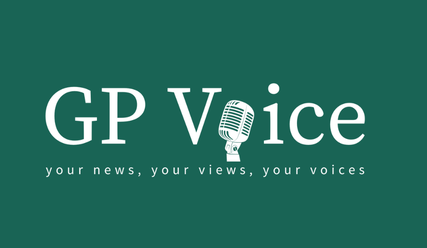
3 April 2025 | GP Voice
April 2025
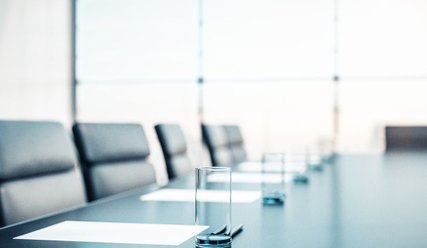
26 March 2025 | College and members
Voting open for the 2025 College election
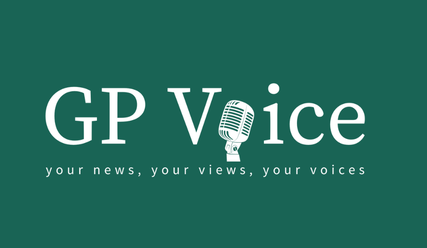
6 March 2025 | GP Voice
March 2025
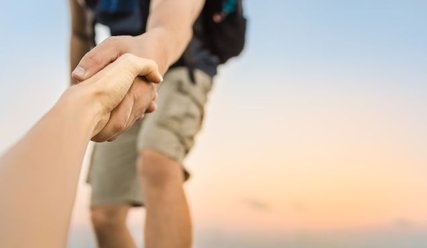
4 March 2025 | Media releases